Synchronisation requirements in URLLC networks
Gain a deeper understanding of URLLC synchronisation and how this affects oscillator choices
5G technology has a focus area known as Ultra-Reliable Low-Latency Communication (URLLC). This technology provides ultra-high reliability and low latency for mission-critical applications that require end-to-end latencies as low as a few milliseconds.
URLLC is essential for Industry 4.0, particularly for factory automation like remote factory operations and machine controls. For example, construction sites can remotely operate excavators or cranes. Additionally, URLLC is critical for certain medical applications such as remote surgery where surgeons perform real-time surgery by operating a robot from a different location to the patient. Autonomous vehicles are another application of URLLC, where connectivity is crucial for a vehicle to have a visibility of 10 seconds into the future when travelling at speeds of 100km/h. And more recently AI applications will greatly increase the demand for low latency.
All these applications require very high reliability and low end-to-end reaction times, which URLLC provides.
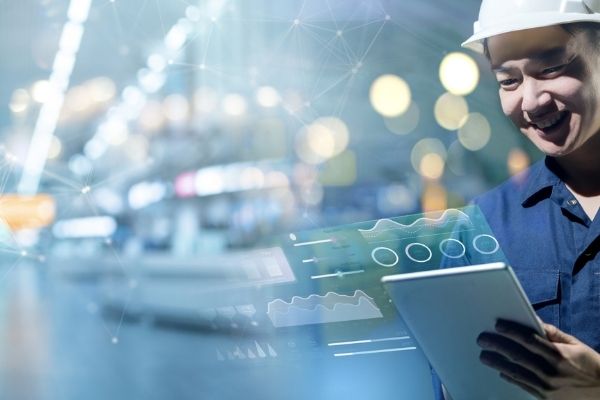
How does URLLC differ from other 5G communications?
Let's take a closer look at the important indicators for a URLLC network. The two main parameters are latency and reliability. Latency is defined as the time it takes for a successful reception to occur, meaning faster reaction times for applications. Reliability can vary depending on the situation, and factors such as distance between transmitter and receiver, visibility, SNR, and packet size can affect it in a cellular network scenario. Other related aspects include throughput, range, connection density, and conditions for establishing a connection. For instance, a surgeon can perform surgery in a moving ambulance because the network is certified to meet the requirements for URLLC.
Latency requirements can range from under 1ms to about 50ms, with critical applications typically requiring 5-10ms. The latest LTE networks can meet latency performance requirements of 20-30ms. Reliability requirements vary, but the most common requirement for critical applications is better than 5 "9s".
Experts suggest that the synchronisation issue is not related to latency but rather the latency variation. However, latency is mainly affected by physical queueing, transmission speed, and protocol design. 3GPP has proposed several mechanisms to address response times and delays, such as requesting scheduling to aid traditional transmission mechanisms and reducing frame size for URLLC to reduce transmit delays.
Additional measures include optimising packet structure for rapid channel information acquisition, retrieving control information, and processing data. Channel coding can be improved, and error correction can be added to reduce code rates and improve channel strength.
Scenario | Latency Target (ms) | Reliability Target (%) |
---|---|---|
Motion Control
|
1
|
99.9999
|
Discrete Automation
|
10
|
99.99
|
Process Automation
|
50
|
99.9999
|
Monitoring
|
50
|
99.9
|
Intelligent Transport Systems
|
10
|
99.9999
|
Tactile Interations
|
0.5
|
99.999
|
Remote Control
|
5
|
99.999
|
As 5G networks continue to develop, the eMBB implementation is the most common starting point. There are two approaches to this: the first involves integrating 5G New Radios with existing 4G networks before transitioning to stand-alone 5G networks. The second approach is a new concept that centralises data centres and replaces BBU functionality with general-purpose hardware, starting with existing 4G LTE radios and eventually replacing them with 5G NR radios.
For carriers to support URLLC, they need a completely new network with superior synchronisation capabilities. This requires hardware support for Carrier Aggregation of all types and guaranteed synchronisation in the backend network to meet various synchronisation requirements.
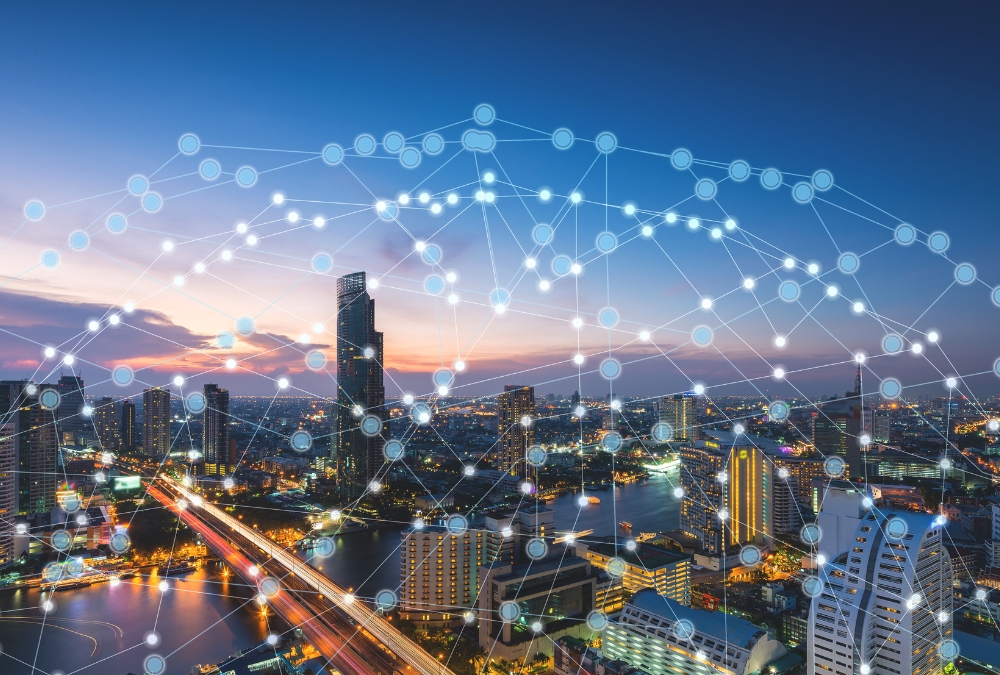
To achieve optimal performance, it is essential to have reliable master clocks and updated equipment clocks. The use of more PRTC-B clocks at the edge has been found to improve performance, as compared to PRTC-A clocks and ePRTCs. It is recommended that the T-GM be placed near the end nodes to minimise the overall TAE from the master to slave. Additionally, it is crucial to fit the T-GMs and edge GMs with holdover capabilities to prevent synchronisation failure from affecting the entire network or services.
One of the main challenges is selecting the appropriate elements to use in the networks. Simply reusing existing equipment may not meet performance requirements. To distribute synchronisation, high-performance T-BCs come in several types, with T-BC type D offering the best performance and minimal TIE. In situations like this, an assisted T-BC architecture is recommended, where GNSS serves as the primary clock, providing minimal network error to the equipment while also offering strong network traceable clock assistance. It is vital to choose clocks with good holdover capabilities to prevent synchronisation disruptions from affecting time-critical applications.
The Radio Unit requires strong synchronisation capabilities, with a 50ppb requirement on the air interface measured across 1-millisecond intervals. The new networks recommend using a physical layer network as a reference for frequency synchronisation, with a strong filter required to achieve the 50ppb frequency accuracy requirement on the air interface.
The selection of oscillators for Ultra-Reliable Low-Latency Communications (URLLC) networks is critical to system design. There are three major reference oscillator parameters to consider:
1. Short to Medium Term Stability
This is represented by the temperature sensitivity of the oscillators. URLLC networks require oscillators with short to medium-term stability (1-100 seconds). The key aspect of this stability requirement is to ensure that the system's temperature sensitivity meets the end equipment's phase variation requirements. A common temperature sensitivity performance for nodes is 0.1 - 0.5 parts per billion (ppb) per degree Celsius. Given the dynamic performance of the nodes, especially end nodes, the filtering requirement is stronger than that for intermediate nodes. A high-performing oscillator is suggested to guarantee the best dynamic behaviour of the system.
2. Holdover Requirement
End nodes should be able to operate independently even when the reference clock fails, and the data plane is available. Higher stability oscillators are needed to support phase holdover of systems. Very stringent phase accuracy requirements are needed to support collaborative features of radios, which are essential for URLLC applications. Considerations for long-term holdover include temperature stabilities of less than 1 ppb, ageing numbers of less than 0.1 ppb, very low random noise generation, low hysteresis, and resonators completely free of micro jumps and activity dips.
3. Lower Phase Noise Requirements
With 5G, low phase noise clocks are needed to support high data rates. Higher reference clock phase noise will result in higher Error Vector Magnitudes (EVM) – a measure of the variation of the constellations from their ideal positions in a phase modulation scenario. This effectively reduces the throughput. The following is recommended EVM limit for modulation levels.
Modulation | Required EVM Limit |
---|---|
QPSK
|
17.5%
|
16 QAM
|
12.5%
|
64 QAM
|
8%
|
256 QAM
|
3.5%
|
1024 QAM
|
1%
|
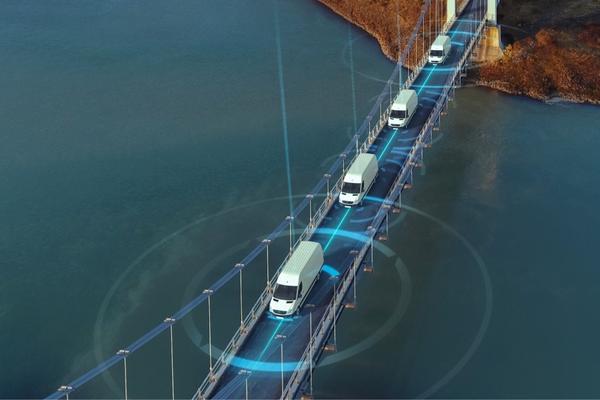
Sub Carrier Spacing | Phase noise - OCXO 122.88MHzdBc/Hz | Phase noise - Ultra Low Noise VCXO 122.88MHzdBc/Hz | Phase noise - Traditional VCXO 122.88MHzdBc/Hz |
---|---|---|---|
1Hz offset
|
-71
|
|
|
10Hz offset
|
-100.3
|
-85
|
-70
|
100Hz offset
|
-125.8
|
-121
|
-100
|
1kHz offset
|
-140
|
-143
|
-128
|
10kHz offset
|
-150.7
|
-158
|
-147
|
100kHz offset
|
-159.3
|
-165
|
-157
|
1MHz offset
|
-160.7
|
-169
|
-161
|
10MHz offset
|
-160.7
|
-169
|
-163
|
EVM Contribution to a 2.4GHz Carrier
|
0.05%
|
0.22%
|
1.38%
|
Summary
To achieve optimal performance, URLLC networks must be built from scratch and equipped with reliable and consistent technology that delivers minimal latency. Some of these networks are deployed in remote geographical locations and demand redundancy services and low latency. To ensure reliability and redundancy in timing networks, synchronisation is of utmost importance and therefore reliable reference clocks are critical. There are numerous innovative frequency reference products that aid in establishing dependable networks with low latency.
If you would like to know more about our Stratum 3E oscillator with 24-hour holdover, visit our PPS Disciplined OCXO page or get in touch with us.
Would you like more content like this delivered to your inbox?
Subscribe to our emails now!
Related content
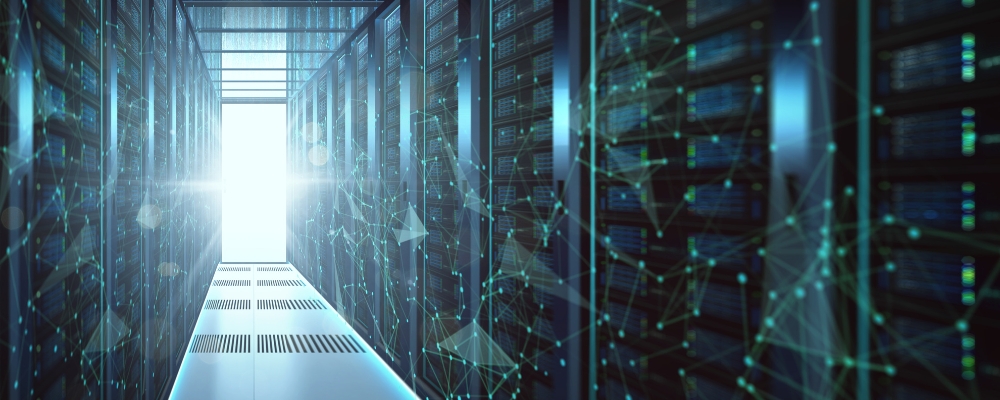
Blog: What is synchronisation holdover, and why is it important in future-proofing telecom networks?
Holdover is a technique used in telecommunications to maintain accurate timing and synchronisation of equipment in the event of a temporary loss of...
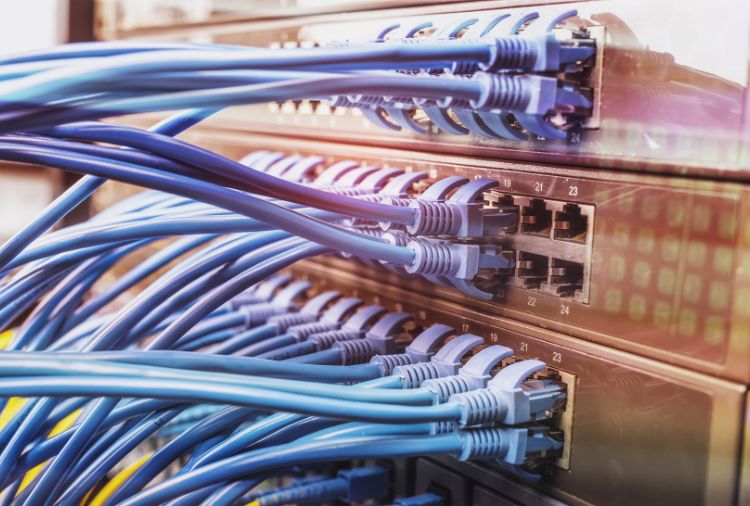
Blog: Frequency reference choices for packet networks
All modern transport networks are based on packet transport technologies. While synchronisation in digital networks has always been important, it has...
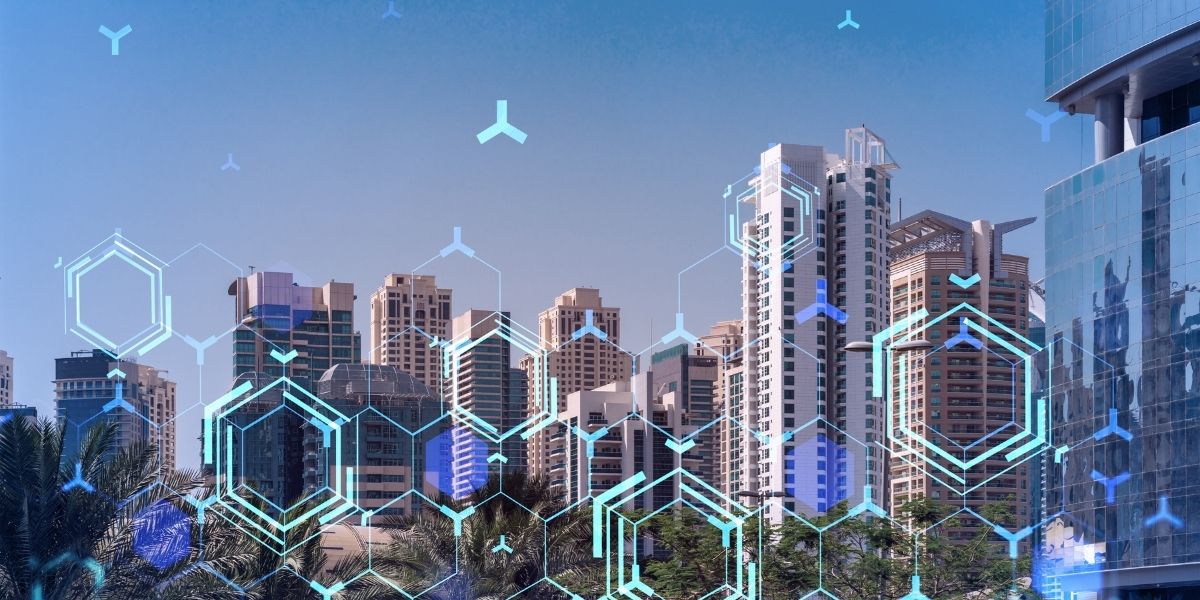
Blog: How to choose oscillators for your 5G small cell deployment
With higher spectral frequencies and shorter reach, 5G networks are much denser than previous generations. Superfast 5G backhaul (mmWave) relies...
Want to know more about our products?
Browse our product pages.
Find products using our parametric search
Talk to our sales engineers