Oscillator basics for telecommunications applications
The very basic building block of digital communication is synchronisation. Synchronisation has many perspectives. In digital transmission, synchronisation is about managing transmission and reception without errors by managing an average transmission and reception rate across nodes. In cellular communication, synchronisation enables the user equipment to work reliably while in motion and when moving from one cell to the next. In advanced networks like 5G, synchronisation is fundamental to the operation and high-performance of the networks.
Digital network synchronisation is hierarchical; an atomic source clock from the core of the network distributes synchronisation across all the nodes. Each node has a servo system that will acquire synchronisation information from the higher level and steer the local timing reference oscillator in the system. Depending on the level of accuracy required by the system, the servo system will average the incoming synchronisation information. The more averaging, the better output accuracy becomes. However, during this averaging time, the output clock is controlled by the local reference. Therefore, for a particular level of output accuracy, a specific level of stability of the local reference oscillator is required.
Oscillators provide the ticks for digital circuits to operate. The ticks progress the digital states and make the digital transitions possible. Oscillating circuitries are made possible using several technologies, of which quartz crystal-based oscillators are the most popular. Quartz oscillators are based on the piezoelectric effect. A voltage is applied to a piezoelectric material, forcing the material to have mechanical deformation. When the voltage is removed, the material gets back into its original form and creates a reverse voltage across it. When this technique is applied across an oscillating circuitry, it creates a sustained oscillation.
For a given oscillator, there are two key parameters to reference; accuracy and stability. The accuracy of an oscillator is the ability to provide a frequency with the same as an ideal absolute reference frequency. For atomic clocks acting as the master sources of time, accuracy is essential. For clocks on the transport equipment, which always receive timing from the upper hierarchy, accuracy is not essential. Stability is the feature of the oscillator to continually produce the same frequency without much change. This feature matches the requirement to work with servo systems, where the system can hold the output frequency or minimal deviation between the correction intervals.
Quartz material is grown in autoclaves, after which wafers are made out of the bars. The blanks are made at different ‘cut angles’, resulting in specific oscillation behaviours. The most popular cut types used in telecommunication equipment are the AT-cut and SC-cut crystals. The blanks are connected with electrodes to pass through electricity and are made into resonators. These are packaged in different ways to produce oscillators of different technologies and performance levels.
Contents
- Introduction to oscillators
- The effects of temperature on short-term frequency variation
- Effects on short-term frequency variation that are not temperature-related
- The accuracy of an oscillator over time determines the lifetime of the oscillator
- How to choose the right oscillator for your telecom application
Quartz crystal-based oscillators can generate the purest sinewaves, mainly containing a single frequency signal. However, there are several environmental effects that cause frequency deviation in quartz material. And these are precisely the specifications you need to be looking at when selecting an oscillator for your application.
Temperature is the single most important factor that significantly affects the quartz oscillators’ short-term frequency variation. A typical oscillator can undergo 20 to 100 ppm frequency variation when temperature changes in the operating range. For example, from -40° to -85° temperature change on a crystal oscillator will show a frequency variation of up to 100ppm. The oscillators’ frequency deviation is generally measured in parts per million (ppm), referring to the amount of deviation that happens with each million Hz. Oscillator frequencies discussed here are usually in the MHz range. Using the ppm unit generalises the variation across frequencies for easy understanding and evaluation. Parts per billion (ppb) is another standard unit used to express the frequency deviation, referring to the change in one billion Hz.
Temperature-compensated Crystal Oscillators (TCXOs)
Different techniques are used to make the quartz oscillator resilient against temperature changes. The first of its kind is Temperature-compensated Crystal Oscillator (TCXO). The Crystals are characterised for their temperature performance, and compensation values are derived while producing the TCXOs. In the field operation, the TCXO device measures temperature seen by the crystal, and the known compensation values are dynamically applied to minimise the frequency deviation. With such techniques, the stability of the oscillator can be improved to 50-100ppb levels across the operating temperature range.
Oven Controlled Crystal Oscillators (OCXOs)
A second method of stabilising the oscillator is using oven control. In this technique, the oscillator is placed in a heated oven to reduce the impact of external temperature changes. Usually, the oven’s temperature is higher than the operating temperature region of the oscillator to reduce the impact of external variations. Such devices are called Oven Controlled Crystal Oscillators (OCXOs).
Voltage Controlled Crystal Oscillators (VCXOs)
Oscillators generally come in two forms: devices with voltage control options to change output frequency and devices with fixed frequencies. Voltage Controlled Oscillators are used in line with analogue implementations of phase lock loops. Fixed frequency devices are generally used to either drive digital systems directly or to drive synthesisers with a digital mechanism to synthesise frequencies. Fixed frequency devices are becoming more popular with increased acceptance of digital synthesis.
Ageing
Ageing is the systematic variation that happens to the oscillator over a while. The oscillators are built on quartz blanks, and the blanks undergo the deformation either by vaporisation or deposition of elements causing width change to the Quartz material. Even though the difference is minimal initially, this variation causes frequency drift over long periods. Usually, such ageing is characterised over one day and expressed in ppb/day variation.
Hysteresis
Hysteresis is the phenomenon of resonator accumulating frequency deviation on temperature cycling. The oscillator’s frequency at a certain temperature point changes depending on whether the point was reached from a higher temperature level downwards or a lower temperature level upwards. This effect causes servo systems to operate incorrectly where high precision is required.
Re-trace
Re-trace is the feature that causes the oscillator to differ in frequency in power recycling. The oscillator will produce a slightly different frequency from one produced before it was turned off. The duration of power-off increases the drift. Re-trace also creates issues with synchronisation performance.
Shock and vibration
Shock and vibration are two other factors that cause the oscillator to deviate in frequency. The quartz crystal blank piece is attached to the electrodes using electric clips, glue, or similar techniques, and the stress release of these bonding systems may also cause frequency deviation.
Phase noise
The phase noise characteristics of an oscillator indicate the power of frequencies other than desired frequency produced by the oscillator. The signal power level at various frequency offsets from the carrier frequency is represented in dBc/Hz in the phase noise diagram. Phase noise is essential in applications where the primary frequency component must be clearly distinguished. One typical example is the phase modulation schemes where the receiver needs to have constellations minimally dispersed as possible.
Other environmental effects
The initial frequency accuracy is defined as the frequency accuracy of the oscillator when the device is powered up on the end application. Most oscillators are calibrated to absolute 0ppm when shipped out of the factory. The transport and storage cause the oscillator to move from its nominal frequency; the most significant deviation generally comes when the oscillator undergoes reflow soldering. The stability levels of oscillators used in telecom equipment range from 300-500ppb.
Other system effects such as power supply variation and load variation also impact frequency drift.
The lifetime accuracy of an oscillator is the worst overall accuracy an oscillator achieves, including all possible variations. Standards define the duration of ‘lifetime’ as 20 years. As the equipment lifetime is getting shortened because of the fast technological advancements, lifetime is now generally accepted to be 10 years by the industry.
In summary, oscillators are susceptible devices, and to achieve the highest performance level, you have to deploy several electrical, thermomechanical and advanced compensation techniques. But if you are not a synchronisation expert, how do you choose the right oscillator?
There are several industry standards that support telecom applications. Oscillator requirements can generally be derived from these standards:
- ITU-T study group 15, Question 13 dealing with network synchronisation and time distribution performance
- O-RAN has specifications for synchronisation architectures and solutions for front-haul timing
- 3GPP for air interface requirements of cellular networks
- OCP-TAP for synchronisation requirements on data centres.
There are other specifications that are not completely covered in the standards yet, but do need to be taken into account for implementation details. If you would like to discuss your specific requirements, get in touch with us.
This guide is based on a tutorial delivered at the ITSF International Timing & Sync Forum 2021 in Brighton, UK.
Would you like more content like this delivered to your inbox?
Subscribe to our emails now!
Related content
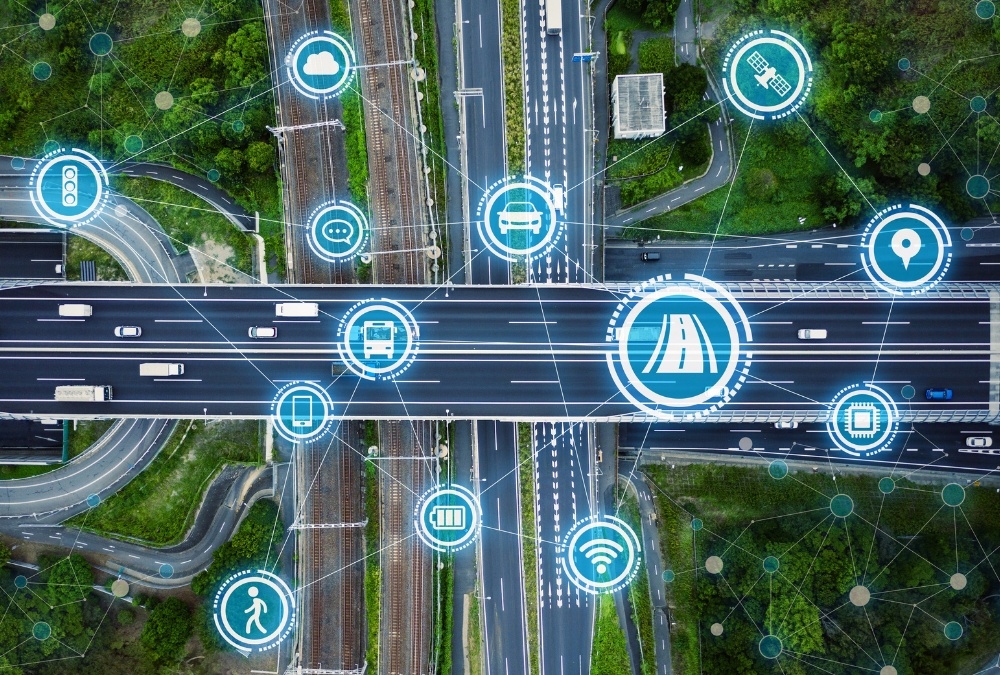
White Paper: Synchronisation requirements in URLLC networks
5G technology has a focus area known as Ultra-Reliable Low-Latency Communication (URLLC). This technology provides ultra-high reliability and low...
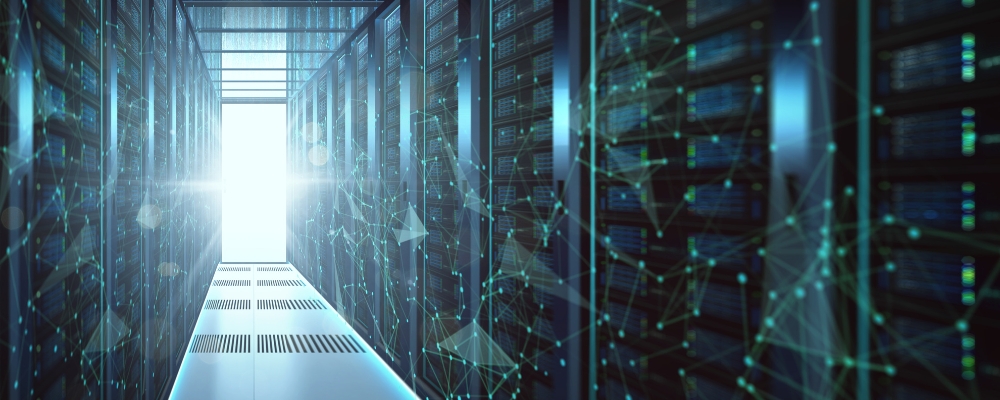
Blog: What is synchronisation holdover, and why is it important in future-proofing telecom networks?
Holdover is a technique used in telecommunications to maintain accurate timing and synchronisation of equipment in the event of a temporary loss of...
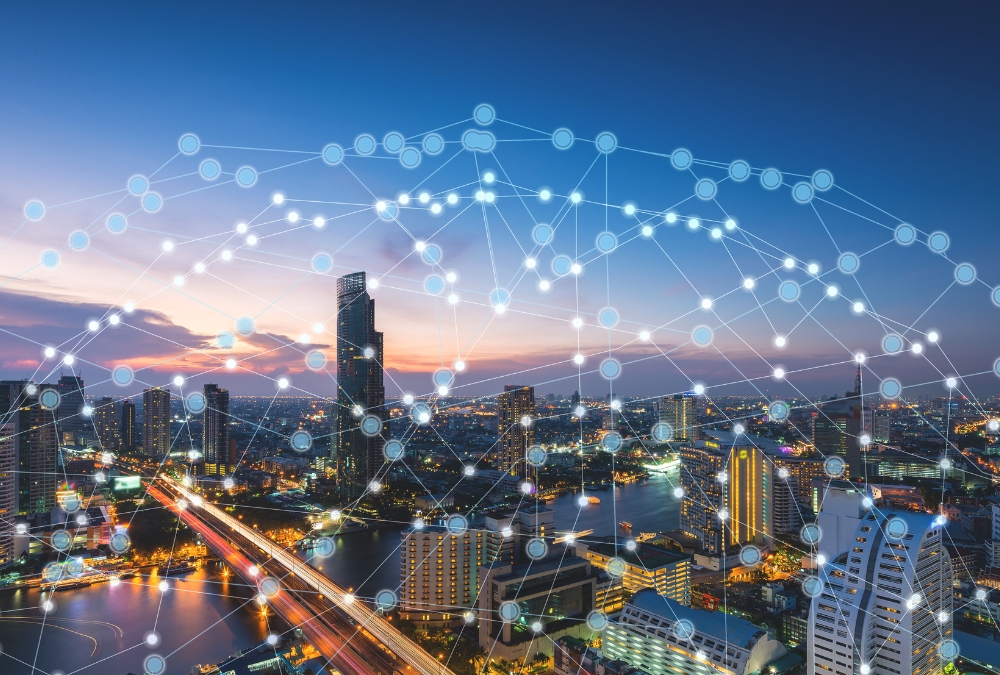
Blog: Synchronisation fundamentals for digital communication systems
Synchronisation is fundamental to most coordinated activities, from a performance by a symphony orchestra or ballet company to digital...
Want to know more about our products?
Browse our product pages.
Find products using our parametric search
Talk to our sales engineers